Cell Division: A Bit Like a Line Dance
- lscole
- Apr 22
- 9 min read
Updated: 1 day ago
Most of the time, most of your cells are simply doing their jobs. Neurons in your brain are sending electrochemical signals. Cardiac muscle cells are contracting rhythmically. Rod and cone cells are detecting the images you're presently seeing on your screen.
Still, though, at the same time, about 1% of your 40 trillion cells (400 billion) are dividing. Certain of your cells are regenerated continuously (for example, bone marrow, blood, skin, intestinal, and hair follicle cells). In addition to these frequently dividing cells, the body also performs cell division to replace damaged or worn out cells.
Cell division is more common when an organism is growing. About 10-30% of a baby's cells are dividing at any given time. Going farther back, we all started as a fertilized eggs, and the developmental path we took from that single egg cell to a multi-celled embryo to a fetus required an estimated 15 trillion cell divisions. So cell division is happening all the time in every human being, more or less depending on the extent to which that person is growing.
Today, I'll be covering two topics related to cell division. In the first part of the post, I'll review the cell cycle: the four-step, roughly 24-hour process the cell engages in when it's dividing. In the second part, I'll focus on just the last phase of the cell cycle, mitosis. In mitosis, two genomes that have already been replicated in a parent cell are pulled apart into two daughter cells. It's an amazing process that I'm likening to a chromosomal dance.
I should note that by convention, the cell that's dividing is called the "parent cell" and the two resulting cells are called "daughter cells." Later I'll also refer to the two chromosomes that exist post-replication as "sister chromatids." Nothing about gender is being implied by these terms. They're simply historical.
The Cell Cycle
Non-dividing cells are said to be in phase G0. Entry into G1, the first phase of the cell cycle, is typically initiated by a growth factor signal (a protein) sent by other cells. Growth factors lock onto receptors on the outer membrane of the target cell. This initiates an intracellular signaling cascade that ultimately induces the expression of the genes required in G1. The most important of these is a gene for a protein called cyclin D, which is the G1 master regulator protein. I'll say more about cyclin D and other cyclins in a moment.
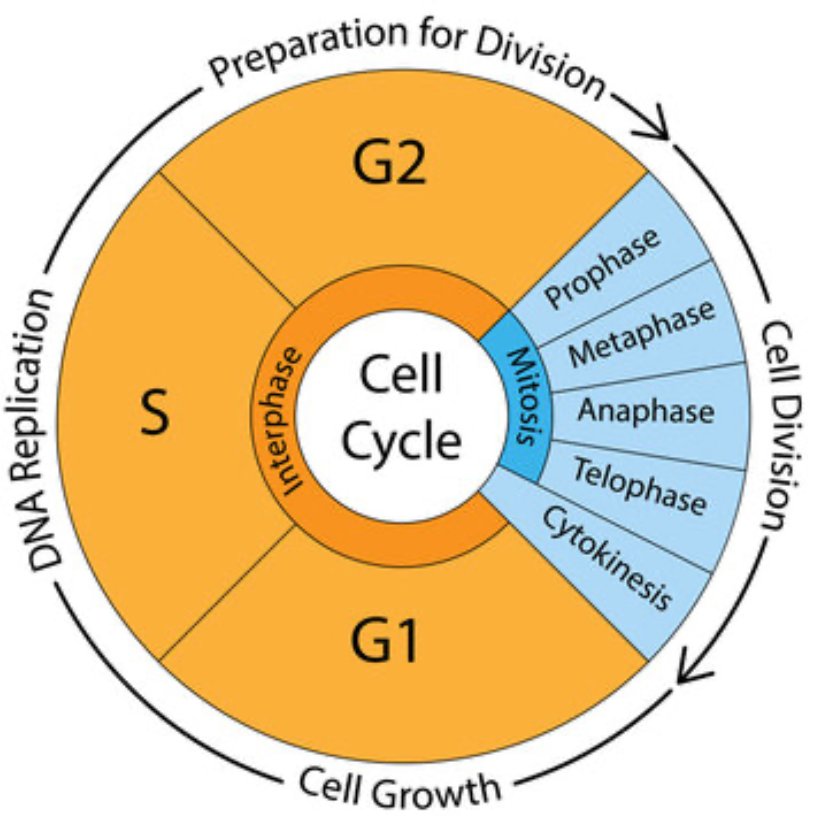
Looking at the figure above, we see that the cell cycle includes a phase where the genome is replicated (called S phase, for "synthesis") and a phase where the replicated genomes are partitioned into the two new cells (usually referred to as M phase, but in the figure just called "Mitosis"). Between these two phases are two gap, or growth, phases: G1 and G2. Let's quickly step through the three phases of the cell cycle that precede mitosis.
G1 is a basic cell growth phase. The first scientists who studied the cell cycle saw nothing happening during this phase and labeled it G1 for "gap 1." Today we know that in addition to growing, during G1 other cell process are taking place. For example, the cell is assessing nutrient availability to make sure it is ready to enter the DNA synthesis phase. And one of the three major cell cycle checkpoints occurs toward the end of G1. At this checkpoint, the cell effectively asks itself (and determines) whether "all systems are 'go.'" A "yes" allows the cell to proceed into S phase.
The synthesis (S) phase of the cell cycle will be the exclusive focus of the second half of this blog. So I won't say much about it here. Big picture, though, the cell's task during S phase is to make a second duplicate copy of its DNA genome. It does so over the course of about 8 hours.
One point I do want to make about S phase is that, following replication, sister chromatids are connected by proteins called cohesins. Cohesin are ring-shaped protein complexes made up of four proteins. During S phase, cohesin molecules are loaded onto DNA and are believed to encircle the two sister chromatids, holding them together like a ring around a pair of wires. So, cohesins don't chemically bond to DNA but, rather, mechanically hold the chromatids together.
Altogether, there will end up being hundreds to thousands of cohesin complexes encircling each pair of sister chromatids. A large number of cohesin molecules connect the sister chromatids at their centromeres. Fewer connect the arms of the sister chromatids. So the cell leaves S phase with the entire genome copied and the resulting sister chromatids attached to each other, especially at their centromeres.
G2 is another growth (formerly "gap") phase. Because our target cell will soon be two cells, it must manufacture more organelles and other cellular components to fill them. That's a core tasks of G2. In addition, during G2 the cell is repairing any DNA damage or replication errors that might have occurred during S phase. The chromosomes that will be the focus of mitosis must be in near-perfect shape before the cell will partition them. There's another key checkpoint at the end of G2 focused on confirming genome integrity prior to cell division.
Cyclins and CDKs
I mentioned earlier that cyclin D is the master regulatory protein during G1. In fact, at each phase of the cell cycle, new genes and sets of genes will need to be turned on and others turned off. Cyclins and their partner CDKs (cyclin-dependent kinases) are master switches and central players in driving the cell cycle. They'll also figure in our discussion of genome replication. So let's pause here and discuss cyclins and CDKs. CDKs first.
A CDKs is a type of enzyme called a kinase. Kinases attach to ATP molecules, remove their last phosphate ion, and place it at a precise location on a specific protein thus causing some specific effect. In fact, CDKs are always floating around the nucleus. But they only function as kinases if they are attached to their particular cyclin partner (they're "cyclin-dependent").
As I mentioned, in G1, cyclin D is expressed. It attaches to two specific CDKs: CDK4 and CDK6. Once those two CDKs are activated, they phosphorylate a specific set of proteins which, in turn, triggers key transitions and processes required during G1, especially the transcription of the genes that code for the proteins that play roles in G1.
Continuing, in the G1 to S transition, cyclin D levels fall and the cell expresses cyclin E, which attaches to and activates CDK2, which then phosphorylates proteins required for the transition. Once in S phase, the level of cyclin E falls and the cell expresses cyclin A, which attaches to and activates CDK2 and CDK1. In G2, it's cyclin A and CDK1. And in M, it's cyclin B and CDK1.
So, again, these cyclin proteins are the master regulators of the cell cycle. They work by activating specific CDKs which then phosphorylate specific places on other proteins, many of which are transcription factors that turn genes on and off.
Mitosis
In S phase, the cell replicated its genome. The cell then passed through G2 and now, in M phase, two copies of the parent cell's 46 chromosomes exist as long, tangled strings in the nucleus. The original and new copy of the genome (the sister chromatids) are connected after S-phase replication by cohesins.
Let's quickly run through the mitosis process before going into the steps in more detail. First, the chromosomes will make their way to an imaginary line across the middle of the cell. Once there, molecular cables will attach each chromosome to bodies called centrosomes at each pole of the cell. The cables will then pull the molecular dancers toward each pole, at which time the cell will pinch in the middle and becomes two cells. Now let's look at these four steps and the cell's starting point, interphase, in more detail.

Interphase: A Jumbled Mess
Again, our starting point is a cell that has just passed through G2 after it replicated its genome during S phase . A human genome has 23 chromosomes. You're born with a genome from each parent, so you have 23 times two, or 46 chromosomes. The interphase (G2) nucleus now contains four complete genomes, or 92 chromosomes all jumbled and intertwined. Think of 92 strands of yarn stuffed into a small knitting bag. The chromosomes can't be distinguished yet visually. Finally, note an important detail in the figure: two small star-like objects float outside the nucleus. These are centrosomes. They'll soon play a key role in chromosome movement.
Prophase: The Puppets Appear
At prophase, the stringy chromosomes are compacted and the centrosomes move to opposite poles. More specifically, the 92 long, intertwined chromosomes in the nucleus are untangled, looped and coiled into compact units by proteins called condensins and cohesins. The cohesins actually hold the arms of the chromosomes (the "chromatids") together. Now the chromosomes are almost in a form that can be pulled into the new daughter cells. We'll call the 46 chromosomes in this form our dancers.
Let's pause to take a look at one of the condensed chromosomes (dancers) and clarify some terminology. When each chromosome was copied prior to mitosis, the original and the copy ended up attached at their middle regions, or centromeres by cohesin proteins. What biology textbooks refer to as "the chromosome" at this point looks like an "X" with the left side being referred to as one chromatid and the right side the other chromatid (sister chromatids).
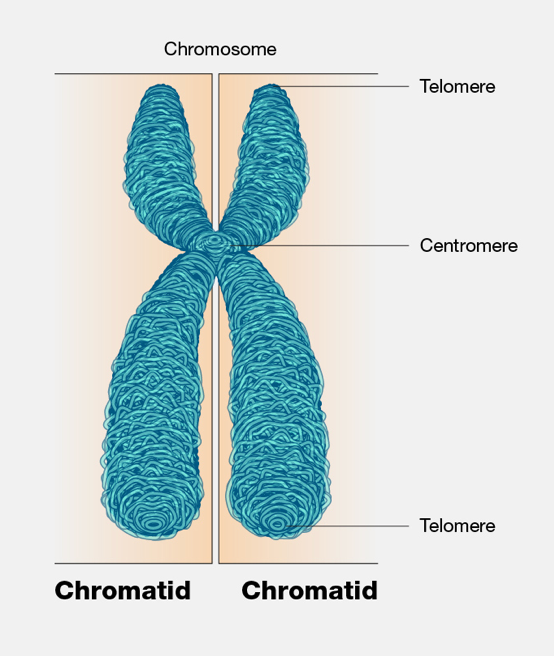
I find this nomenclature confusing so let me clarify. The two left-hand arms of the "X" (called a chromatid in the figure) are actually one complete chromosome and the two right-hand arms are another complete chromosome. So what I referred to in the last paragraph as "the chromosome" (the "X" shaped object) was really two identical chromosomes connected in the middle. So, bottom line: the 92 chromosomes actually take the form of 46 of these X-shaped bodies. Going forward I'll use both terms--chromatid and chromosome--but I'll make it clear to what I'm referring.
Back to prophase. As I mentioned, in addition to the chromosomes condensing, the centrosomes move toward opposite ends of the cell! The two centrosomes are connected by molecular cables (microtubules) that, in this context, are called mitotic spindles. By the end of prophase, the centrosomes will have made it to opposite ends of the cell with mitotic spindles emanating from each and in some cases connecting the two.
Two final acts in prophase: (1) the nuclear membrane breaks down, spewing membrane pieces around the cell. This will also make the chromosomes accessible to mitotic spindles in metaphase, and (2) protein complexes called kinetochores attach to the centromeres. Kinetochores will serve as molecular hooks for mitotic spindles in metaphase.
Metaphase: The Dancers Line Up
The 46 X-shaped chromosomes now line up next to each other at the center, or "equator," of the cell. The centrosomes--which are now at their ultimate destinations at opposite ends of the cell--send out more mitotic spindles that will attach to these kinetochores. By the end of metaphase, all 46 "chromosomes" (92 chromatids) will be aligned along the equator and each of their kinetochores will be attached to a mitotic spindle from one or the other of the centrosomes.
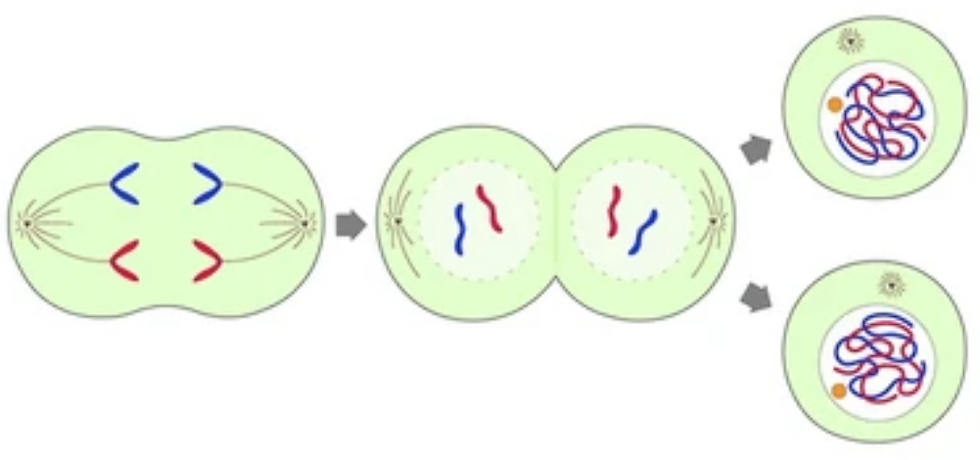
Anaphase: The Dance Begins
At the beginning of anaphase, the cohesin proteins holding the two chromatids together are cleaved by the enzyme separase. How does this work? It turns out that separase has been present in the nucleus since the beginning of the cell cycle. But it has been rendered inactive by the binding of the securin protein. CDK1 also phosphorylates separase prior to anaphase, rendering it inactive. Then, at the outset of anaphase, securin proteins are marked by another enzyme for degradation. And once the securins are destroyed, the separase enzymes become active and cleave the cohesins at a specific place on the protein complex.
The chromatids (chromosomes) are now capable of being separated. Next, they are pulled toward the centrosomes by a process in which the microtubule mitotic spindles shrink little by little. At the same time, other mitotic spindles that connect the two centrosomes do the opposite: they grow, lengthening out the cell.
Telophase: Two New Dance Troupes Form
Two things happen in telophase. First, fragments of the nuclear membrane broken down at the end of prophase are recycled to create two new nuclear membranes around the chromosomes in the daughter cells. Second, the cell pinches inward at the equator. Cell parts (e.g., organelles, macromolecules, metabolites and ions) will be equally distributed around the parent cell such that when the cell does pinch off completely into two daughters in a process called cytokinesis, each will have everything it needs to function. Finally, the compacted chromosomes de-compact in their new nuclei. This completes cell division.
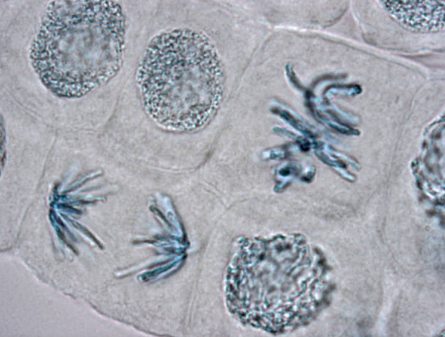
Mitosis--everything we've just been discussing--occurs immediately after genome replication. Hopefully, this review of mitosis puts genome replication in a more vivid context.
Komentáře