Proteins: Flexing Your Muscle Cells!
- lscole
- 2 days ago
- 5 min read
Updated: 17 hours ago
In this post I present an example of ATP-driven protein motor movement. ATP is a frequently employed driver of protein movement in all living cells. The goal here is to absorb a general concept that we'll see several more times as we learn about genome replication.
Specifically, today, we'll look at myosin, a protein complex found in skeletal muscle cells. We'll see what, exactly, makes myosin move (spoiler alert: it's ATP) and how minute movements at the molecular level in muscle cells generate macroscopic skeletal muscle contractions.
My approach here will be to zoom into our target (myosin) level by level. We'll first discuss the muscle cells that comprise muscles. A note: muscle cells are somewhat misleadingly called "muscle fibers." I'll be consistent, though, and use the term "muscle fiber" instead of "muscle cell" in this post.
Next we'll examine structures inside muscle fibers called myofibrils that contain arrangements of mainly two proteins (myosin and its partner actin) called sarcomeres that operate as units of microscopic molecular contraction. It is the movement of many sarcomeres that causes skeletal muscle contractions. Sarcomere movement is ultimately due to the movement of myosin. Our eventual focus--the lowest level of our analysis--will be on how ATP makes the myosin protein move.
Muscles fibers
Skeletal muscles are composed of muscle fibers. Again, these are muscle cells. A warning, though. These are highly specialized cells that don't conform to the picture of the cell that I've drawn so far. To begin with, muscle fibers are extremely long--typically 1 to 5 cm long, but they can reach 30 cm (about a foot long!).
Second, because they are so long, they require multiple nuclei. Each nucleus services the region of the muscle fiber near it. But don't be thrown off: multinucleated cells are the exception rather than the rule. The majority of cells in the human body have one nucleus.
Third, muscle fibers contain many, many mitochondria. A typical human cell has hundreds of mitochondria. Muscle cells contain thousands--up to 8,000--mitochondria. The reason? Muscles require a lot of energy. Mitochondria provide that energy in the form of the ATP molecules they generate by participating in the breakdown of glucose and other sugars.
Finally, muscle fibers have a modified cell membrane called a sarcolemma that allows the fiber to respond to nerve impulses instructing the cell to contract. (see figure below, top drawing).

Myofibrils
Muscle fibers are packed with myofibrils, long, rod-like structures that run the length of the muscle fiber (top and middle drawing in the figure) Myofibrils are not organelles; they have no membrane. Rather, they are classified as cytoskeletal structures. Thye're made up primarily of two kinds of proteins: myosin and actin. These two proteins are arranged into contractile units called a sarcomeres, which we'll discuss next.
Sarcomeres
Myofibrils are made up of repeating units, or building blocks, called sarcomeres (middle drawing in the figure). Sarcomeres, in turn, consists mainly of a thick filament, which is made of many long myosin molecules, and a thin filament made up of two long actin molecules twisted around each other (twisting not shown in figure). Also note that each myosin molecule in the thick filament has a "head" structure (the round extensions that look like lollipops).
Thick filaments are permanently attached to a central wall made of structural proteins called the M line, extending outward in both directions from the M line. Similarly, the actin thin filaments are permanently attached to two outer walls also made of structural proteins called Z lines. One thin filament extends inward from the left Z line and one extends inward from the right Z line. Very importantly, the actin thin filaments interlace between the myosin thick filaments and the two are in close proximity.
It is the interaction between the myosin molecules in the thick filament (specifically, the heads) and the actin molecules in the thin filament that results in sarcomere contraction. And it is the sum of many sarcomere contractions that cause skeletal muscle contractions. But why does the sarcomere contract?
Look closely at the bottom drawing in the figure. It shows a sarcomere in a relaxed state and in a contracted state. In the relaxed state, the round heads of the myosin molecules lie flat against the thick filament. Compare that to the contracted state, where the heads stick straight out from the thick filament.
In the transition from the relaxed to the contracted state, the myosin heads have, in effect, swept back toward the M line. And when they sweep inward, they grab the actin thin filaments and pull them inward toward the M line with them. This pulls in turn pulls the two Z lines inward toward the M line. Voila, a sarcomere contraction!
I think of the interaction between ithe myosin heads and the thin filament as a kind of cyclic rowing motion. It is this repeated rowing motion of the heads that pulls the Z lines toward the central M line that makes the sarcomere contracts. It's like the actin thin filaments serve as a molecular tracks and the myosin molecules serve as molecular motors.
Myosin
Remember, though, that the main objective of this post isn't to show how sarcomere contraction causes skeletal muscle contraction. It is to show how ATP causes myosin head movement. That's where we're going next (and last).
Remember from my post on ATP that when the third phosphate is removed from an ATP (adenosine triphosphate) turning it into an ADP (adenosine diphosphate) and a lone P (phosphate) the result is a burst of energy. I likened this energy burst to a compressed spring being released, or to a small explosion. The point is that just as a spring being released and an explosion can cause movement on our macro-scale, the transition from ATP to ADP + P can cause molecular movement.
Let's dive deeper and go step-by-step through myosin head movement. Follow along in the figure below (Note: Don't be confused by the use of the abbreviation "Pi" in the figure. It's the same phosphate that I'm calling just "P".)
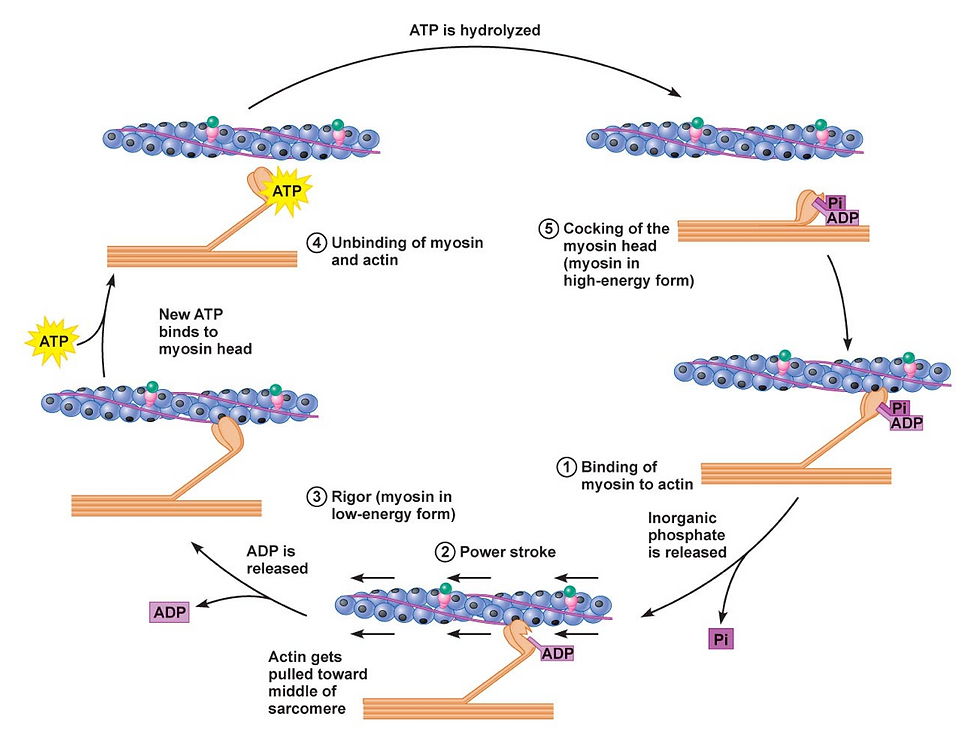
Step 1. Binding to actin. We start with the myosin head attached to the actin thin filament. This occurs when ADP and P are attached to the head following ATP cleavage.
Step 2. Power stroke. P leaves the myosin head first. When it does, it releases the cocked myosin head, which then performs the power stroke, pulling the actin thin filament toward the center M line and causing sarcomere contraction..
Step 3. ADP release. Soon after the power stroke, the ADP molecule is released, leaving the ATP/ADP binding site vacant and awaiting the arrival of an ATP molecule. The myosin head is still attached to actin.
Step 4. ATP binding. When ATP does arrive, it causes the detachment of the myosin head from the already pulled actin thin filament.
Step 5. ATP cleavage (energy used). When the ATP is cleaved into ADP and P, it's as if an explosion or a released spring cocks the myosin head, putting it position for the next power stroke. The resulting ADP and P remain attached to the myosin head, and we cycle back to Step 1.
So, in the case of myosin head movement, the cleavage of ATP does not actually cause the power stroke. Instead, it cocks the myosin head so that when the cocked head is released upon the release of P, the power stroke occurs.
Every time you move a muscle, millions to billions of myosin head cockings and uncockings, and sarcomere contractions occur. Again, we see that we are nothing more than our cells. Macroscopic muscular movement are simply the sum of microscopic sarcomere movements inside of myofibrils inside muscle fibers.
Comentarios