DNA Packaging
- lscole
- May 2
- 7 min read
Updated: 10 hours ago
Two human genomes adding up to 6 billion base pairs are contained in the nucleus of every cell in your body. Different kinds of cells use different genes. That depends on the cell’s job. But every cell contains the whole genome, or every human gene.
One of the cell's challenges is that the genome is very large but its nucleus is very small. If we could stretch out the human genome--in other words line up the 6 billion base pairs end to end--it would stretch nearly 6 feet! Yet the nucleus of a typical cell is only about 5-µm in diameter (5 x 10-6 m). This is 10,000 times smaller than a dot of ink from a ballpoint pen. Six feet of DNA in a container 10,000 times smaller than an ink dot. Mind boggling.
How does the cell do it? To begin with, to say that DNA is thin would be an understatement. Its diameter is 2-nm (2 x 10-9 m), which is smaller than a wavelength of visible light. (This makes visualizing DNA with a light microscope impossible.)
Second (and the topic of this post): the genome is highly packaged and carefully packaged. Consider a spool of thread or a skein of yarn. To make long stringy things fit into small spaces we wind them up... often around some kind of spool So, the answer to the question of how cells do it: DNA is both very skinny (several atoms wide) and very tightly packaged.

As with everything that happens in the cell, genome packaging involves many proteins and protein complexes acting in a choreographed manner. Human genomic DNA undergoes four levels of packaging. Let's review them quickly first before getting into the details.
(1) DNA is wrapped around complexes of eight proteins (histones) that act like little spools. The resulting entities are called a nucleosomes. While naked DNA has a diameter of 2-nm, nucleosomes have a diameter of about 10-nm (see figure below).
(2) The nucleosomes are further coiled into a helical structure called a 30-nm helical fiber.
(3) The 30-nm fiber is looped out from a protein scaffold to create a 300-nm structure.
(4) The 300-nm structures are further condensed to the mitotic form of the chromosomes. These are roughly 700-nm in diameter and are the chromosomes as we often see them--for example, in the karyotype photo at the beginning of this post.
Thus, the cell starts with a strand of DNA that's 2-nm in diameter and ends up--after four levels of packaging--with a chromosome 700-nm in diameter. Let’s look at each packaging step in more detail.
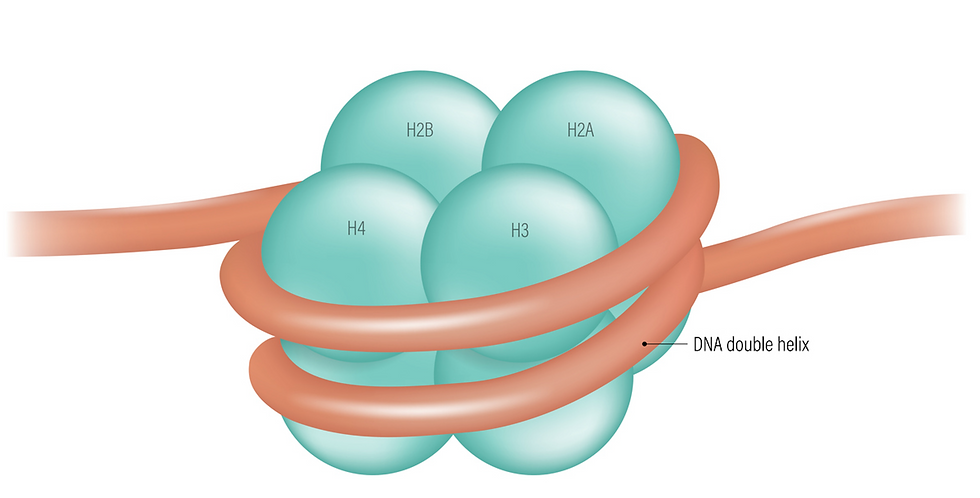
The first level of packaging (nucleosomes) results in a form called beads-on-a-string. Two each of four different histone proteins (H2A, H2B, H3, and H4) create a molecular spool that has the shape of a flat disk or short cylinder (see figure). Other histone chaperone proteins (FACT, ASF1, and CAF-1) carry the eight histones to the DNA and assemble the cylinders.
In a nucleosome, approximately 150 nucleotide pairs of DNA wrap around each cylinder. Spanning the gaps between nucleosomes are naked DNA linkers that are a few to about 100 nucleotide pairs long. The nucleosomes and the interspersed linkers create the impression of beads-on-a-string (see photo).
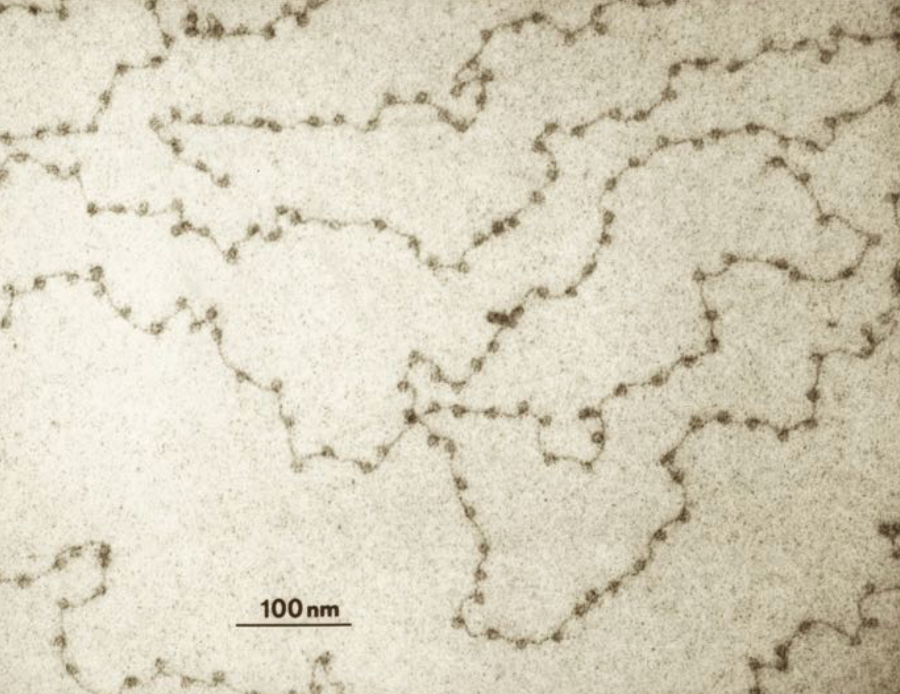
The beads-on-a-string form of DNA has other proteins (not histones) attached to it that we’ll discuss shortly. But the combination of DNA, the histone spools, and these non-histone proteins is called chromatin. Chromatin seems to self-assemble. DNA is negatively charged. Histones are positively charged. This helps their coalescing into nucleosomes.
Surprisingly, nucleosomes are not stagnant things. They move along the DNA. In fact, each unwraps about four times per second, remaining exposed for about 10-50 milliseconds at a time. Also, non-histone proteins including chromatin remodeling complexes push nucleosomes down DNA. So the "beads" are constantly moving, making the DNA available for protein binding during gene expression, DNA repair, and genome replication.
But beads-on-a-string DNA (that is, in a form that isn't further packaged) is not often found in the cell. The naked DNA linkers are too susceptible to breakage and cleavage. Instead, there is a second level of packaging in which the nucleosomes are packed on top of each other. This results in a helical array called a 30-nm helical fiber (see figure below).
How do these 30-nm helical fibers form? It is not well understood but some things are known. First, the histone proteins I mentioned before (H2A, H2B, H3 and H4) are globular proteins, but they all have long polypeptide tails (not shown in the figure). The tails are needed for the 30-nm helical fibers to form. (We will soon see that they also play a key role in controlling additional aspects of chromatin structure and function.) A fifth histone protein (H1, also known as the linker histone) is also critical for the helical structure to form.

Next, in a third level of packaging, the 30-nm fiber is looped out from a central scaffold of proteins to create a 300-nm structure. In fact, the loops can range from tens of thousands to hundreds of thousands of base pairs.
It is at this level of packaging that the cell seemingly makes decisions about what stretches of DNA need to be most accessible for transcription. Loops are the regions of the genome that will need to be accessed by this particular cell.
Like nucleosomes, the 300-nm structures are dynamic, changing as the cell’s developmental stage changes and/or different genes need to be expressed. Loops form and then disappear in favor of new loops.
So, the looping pattern adopted by the cell impacts gene activity. But there are other aspects of packaging that I haven't mentioned yet that also greatly impact gene expression. Most notable is histone modification. This leads to the notion of a histone code.
The cell contains enzymes that attach a wide variety of chemical modifications mainly to the tails of the four core histones. The cell also has enzymes that remove these modifications. The chemical modifications sometimes have a direct impact on the DNA—for example, by altering the way the DNA wraps around the histones, perhaps to make the DNA more accessible. More often, the attachments play an indirect role. They serve as signposts that aid in the recruitment of other enzymes or enzyme complexes. The signposts tell proteins to do their job (be it transcription, chromatin remodeling, DNA repair, etc.) at this now identifiable site along the DNA.
That also means that there are proteins in the cell that recognize either single modifications on histones or multiple nearby histone modifications and that know what to do to or with the DNA at that site. Again, it might be that a gene needs to be transcribed. Or it might be that there's an error in the DNA that needs to be repaired. Or it might be that that stretch of DNA is ready to be replicated. Scientists are studying what each signpost or set of signposts means inside the cell. They are trying to decipher the histone code.
How does the cell know which histones to modify in the first place? This, too, is an area of active research. In some cases, protein transcription factors that attach to DNA sequences near genes recruit histone-modifying enzymes. In other words, as genes are turned on and off, chromatin remodeling complexes are brought to specific regions of DNA where they act locally to influence chromatin structure.
Other influencers of chromatin access are variant histone proteins (i.e., histones that are slighlty different than the four core histones (H2A, H2B, H3 and H4) and the linker histone (H1). The cell sometimes swaps out the standard histones and replaces them with these variant histones. Nucleosomes containing variant histones are more available to the cell’s transcription machinery.
Finally, the last point of control with respect to DNA access is chromosomal territories. Within the nucleus of a cell, certain chromosomes in their 300-nm looped out form occupy certain regions in the nucleus. These are chromosome territories. It has been noticed that within these territories, DNA containing unneeded genes tends to be closer to the nuclear membrane and those that will be expressed tend to be closer to the center of the nucleus.
Taking this all in, what we see at the third level of packaging is an unbelievable dance of proteins and DNA. The nucleosomes in the beads-on-a-string are constantly opening and moving along the DNA. The loops in the 300-nm fiber frequently open and close. Protein enzymes are placing specific chemical signposts on specific histones to target them for different cellular processes—most notably, gene expression. Other proteins are floating around the nucleus, searching for individual signposts or groupings of signposts that tell them where to take action. The nucleus is a very dynamic environment.
The fourth level of packaging only occurs during mitosis (i.e., cell division) and specifically during metaphase when the chromosomes are about to be pulled into each of the two duaghter cells. This is the most compact form of DNA. To get there, the 300-nm loops (which include the 30-nm helical fiber) are supercoiled. The 700-nm form of the chromosome is a coiled coil. Whereas the 300-nm looped structures are accessible for transcription the 700-nm mitotic chromosome is not. Gene expression is necessarily stopped during metaphase.
So there's DNA packaging. Somehow the cell knows both when and how to pack (and unpack) its DNA depending on its needs. Many, many different protein are called into action (expressed) in order to: (1) serve as nucleosome cores, (2) assemble the nucleosome cores from 4-5 different proteins, (3) wrap the 10-nm beads-on-a-string up into perfectly stacked 30-nm helical fibers, (4) loop the helical fibers out from a scaffold of proteins to create a 300-nm fiber, (5) make sure that the loops at the 300-nm level correspond to genes that will be needed by this particular cell, (6) Further condense the 300-nm stractures into their final form: the 700-nm mitotic form of the chromosomes that are so compacted they can be pulled through the cell by a mitotic spindle without interrupting any other chromosomes.
This quick list still leaves out many details on how all this occurs. We'll dive deeper into molecular details in general when we discuss genome replication.
Comments