Proteins: Tiny Machines
- lscole
- Apr 9
- 6 min read
Updated: 3 days ago
A 30,000-foot view. Proteins account for about 50% of the cell's weight. That's much more than DNA, which accounts for 1-2%. It's also more than RNA, which accounts for about 20%. So--big picture--about half of the cell is proteins. But it isn't just the quantity of proteins that makes them important. Proteins are the workhorses of the cell. They do almost everything.
In this blog, I'll be categorizing proteins in two different ways. The first and most important is based on the protein's purpose: some proteins play functional roles (they do things in the cell) and others play structural roles (they support things in the cell).
The best-known functional proteins are enymes--proteins that speed up chermical reactions. But there are other kinds, including signaling proteins, receptors, transcription factors and transport proteins, all of which I'll talk about shortly. I'll be focusing much more attention on functional proteins in this blog, since they'll be the main players in genome replication.
At the risk of offending some other molecules, think of the structural proteins as simple building materials. They provide mechanical support to the cell overall and also scaffold organelles. Structural proteins aren't central to our story about genome replication.

The second protein categorization scheme is based on shape. Many proteins have a roughly round or irregular shape. These are globular proteins. Most, but not all, are functional. Other proteins are longer or more cable-like. These are called fibrous proteins and most, but not all, play structural roles.
Back to 30,000 feet. It's not just that the amount of protein in a cell that's impressive. And it's not just the fact that they're the cell's "do-ers." It's also impressive that human cells have the ability to express tens of thousands of different proteins, each with a unique three-dimensional shape. Much of the diversity of proteins is due to the many kinds of functional proteins (15,000-20,000). There are fewer kinds of structural proteins (1,000-2,000).
In the cell analogy I used a few posts ago, I likened functional proteins in a cell to workers in a factory. But how do proteins do their jobs? It's simple: it's based only on shape. I generally hesitate to make absolute claims, but I think it's safe to say that everything that happens in a cell is based on shape. Molecules don't use words. Their language is shape.
Below are examples of diverse functional proteins doing their jobs. Note the importance of shape in each example.
Enzymes: Some globular proteins have a nook that a specific smaller molecule fits into that facilitates a specific chemical change. Proteins that speed up chemical reaction are called enzymes. For example, in the first step in glycolysis (the breakdown of glucose) the enzyme hexokinase transfers a phosphate group to a glucose molecule. That's all it does. But it does it well!
Signaling proteins: There are also signaling proteins. These proteins typically travel in the blood and then attach to receptors (also proteins) on the surface of specific cells to, in effect, "tell" them to behave in a certain way. For example, insulin is a protein hormone produced by the pancreas. It travels in the blood then attaches to cellular receptors, thus signaling them to absorb glucose.
Motor proteins. Imagine a fibrous protein capable of pulling itself along a second fibrous protein to effect movement. This is what the motor proteins actin and myosin do. As many actin-myosin complexes in your voluntary muscle cells slide along each other in a contracting motion, it causes muscle fibers to contract at the macro level.
Transport proteins: Other proteins are involved in transporting nutrients and other small molecules around the body. For example, hemoglobin proteins floating freely in the blood carry oxygen molecules to every part of the body. Other transport proteins carry specific molecules through the cell membrane either into or out of the cell.
Transcription factors: As we'll see, genes coded in our DNA provide the information (and shape) needed to make proteins. But there's a bit of a circularity here because it is proteins that turn genes "on!" Proteins that attach to DNA near a gene's starting point and thereby activate that gene are called transcription factors. We'll cover transcription soon. For now just be aware that it kicks off protein synthesis inside the cell.
Carrier proteins: Another kind of transport proteinworks inside the cell carrying large structures. One of these proteins is kinesin. Kinesin literally walks (yes, it has two "legs") along stretches of long microtubules (components of the cytoskeleton) carrying large vesicles, organelles, and molecular complexes to other parts of the cell where they are needed. Here's an animation showing a kinesin protein carrying a vesicle.
As you can see, taken as a group, proteins perform a diverse set of jobs in the cell. But each individual protein performs a very specific job (or very small number of jobs).
How does the cell create thousands of different protein machines? Efficiently! Proteins are built from just 20 different subcomponent parts called amino acids. Amino acids are all chemically diffferent from each other, but they are similar in that they all capable of attaching to each other in linear fashion. On average, a human protein might be composed of 300-400 lined up amino acids. But proteins can range in size from 50 amino acids to thousands of amino acids long.
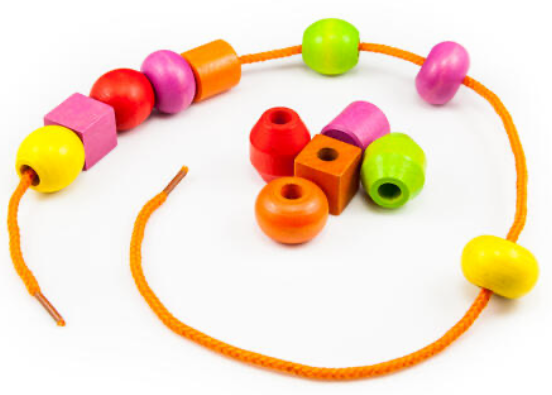
Think of 20 differently colored beads that you're threading on a string. In doing so, you can put any number of beads on the string in any order. Each of the differently colored beads might represent a different amino acid. So stringing the beads is a bit like creating a polypeptide, or protein. At its most basic, a protein is a linear stretch of amino acids. We call the protein's linear order of amino acids its primary structure.
In the previous paragraph, I used the term "polypeptide." The attachments between amino acids are called peptide bonds. Another term that's used in discussing proteins is "polypeptide," meaning "many peptide bonds." A "polypeptide" is simply a linear string of amino acids. It doesn't yet "work." A "protein" is a polypeptide, but one that has folded on itself and is now functional.
Importantly, different amino acids have different chemical properties. Some are positively charged; others are negatively charged. Some are large; some are small. Some are hydrophilic (attracted to water); others are hydrophobic (repelled by water). Thus, each of the 20 amino acids responds to other amino acids in their environment differently. Because of all the attractions and repulsions between different amino acids, linear protein molecules fold into a three-dimensional shape.
I want to repeat this: because of each amino acids' distinct properties--some attract, some repel, some are larger and others are smaller--a protein's linear primary structure always folds up on itself. In what we can think of as the first step in folding, two very common three-dimensional secondary structures appear. These are "alpha helices" (a single helix, not a double helix like DNA) and "beta pleated sheets" (formed when a run of amino acids forms switchbacks with itself).
Don't worry about the names. The point is that in a water-based milieu, the linear array of amino acids (primary structure) will tend to quickly fold into two common three-dimensional structures (secondary structures) . Neither of the two kinds of secondary structure are required to be present in a given protein. But one or more of both often are.
After the alpha helices and beta pleated sheets form, the protein continues to fold on itself such that the two kinds of secondary structures get positioned within the larger folded structure. This is referred to as the protein's tertiary structure. This is the functional three-dimensional form of proteins--forms capable of performing jobs such as serving as enzymes, signaling, carrying molecules around the body, contracting muscles and turning on genes.
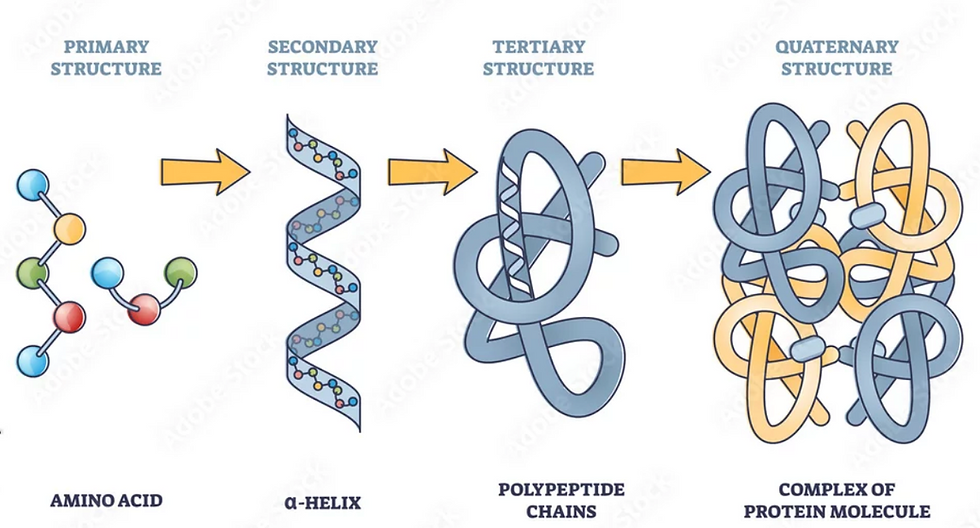
Finally, in some cases, multiple folded polypeptide chains (i.e., proteins) assemble to form a multi-protein complex. When more than one protein combines into a complex, the complex is an example of quaternary structure. Quaternary structures can contain two, three, four, or five proteins and would be referred to as "dimers," "trimers," "tetramers," and "pentamers," respectively. The oxygen-carrying hemoglobin molecule I mentioned earlier is a tetramer comprised of two copies of two different polypeptides known as alpha and beta chains. Each of the four polypeptides can delivering one oxygen molecule, but only in the context of a tetramer complex.
That's a quick introduction to proteins. As we get farther into our discussion of genome replication, we'll see that innumerable functional proteins play the leading roles. Next, let's talk about DNA!
Comments